Studying Star and Planet Formation from The Stratosphere
In my research, I study how magnetic fields influence both star and planet formation. In particular, I design, build, and fly stratospheric balloon-borne polarimeters, which by operating above 99.5% of the atmosphere can map the magnetic fields of star-forming regions, and I then follow-up my observations with powerful ground-based telescopes.
I am also designing and build new types of balloon-borne telescopes. Currently, my group is leading the Balloon-borne Very Large Baseline Interferometry Experiment (BVEX), a project to demonstrate that balloon telescopes can be used in Earth-sized networks of radio telescopes. These balloon interferometer stations should be able to produce ultra-high resolution images when paired with large ground-based telescopes hundreds to thousands of kilometers away.
How do stars and planetary systems like our own solar system form?

Stars form out of vast clouds of molecular gas. However, this process is incredibly inefficient, in that typically at most a few percent of the gas in molecular clouds will end up in stars. Why is star formation so inefficient, and what physical processes set the properties of the stars and planetary systems that form in molecular clouds? Answering these questions is crucial to understanding everything from the formation of our solar system to the chemical evolution of our universe.
To understand star formation we need to study the physics that regulates this process: the gravitational attraction between the gas particles, supersonic shocks and turbulent gas motions, feedback from young stars that can ionize and destroy their parent molecular clouds, and the magnetic fields that permeate molecular clouds. Of these magnetic fields are the hardest to observe, and therefore the least understood.
Do magnetic fields reduce the efficiency of star and planet formation?

Star-forming molecular clouds are threaded by magnetic field lines. If the cloud magnetic field is strong and well coupled to the gas then it is much easier for the gas to move parallel to the magnetic field lines rather than across field lines. In this way magnetic fields can slow down, or perhaps even stop the gravitational contraction of gas clouds into stars, perhaps partially explaining why star formation is so inefficient.
But are magnetic fields in molecular clouds actually strong enough to slow down star formation? We don’t really know because it is extremely difficult to directly observe the strength of magnetic fields in molecular gas. However, we can use the fact that dust grains in molecular clouds tend align perpendicular to their local magnetic field. When these extremely cold dust grains glow they emit far-infrared or sub-mm light that is linearly polarized in the direction perpendicular to the magnetic field lines. We can therefore use this polarized radiation to map out the magnetic field of distant star-forming regions.
Unfortunately most far-infrared and sub-mm light is absorbed in the Earth’s atmosphere. Even worse the atmosphere emits light at those same colours, making it very difficult to observe polarized light from the star forming regions in our galaxy. To map magnetic fields we either need to look at even redder light (where the glow from dust grains is much fainter and harder to detect), or build a polarimeter that can somehow get above most of the atmosphere.
Stratospheric balloon-borne telescopes: an inexpensive ticket to near-space
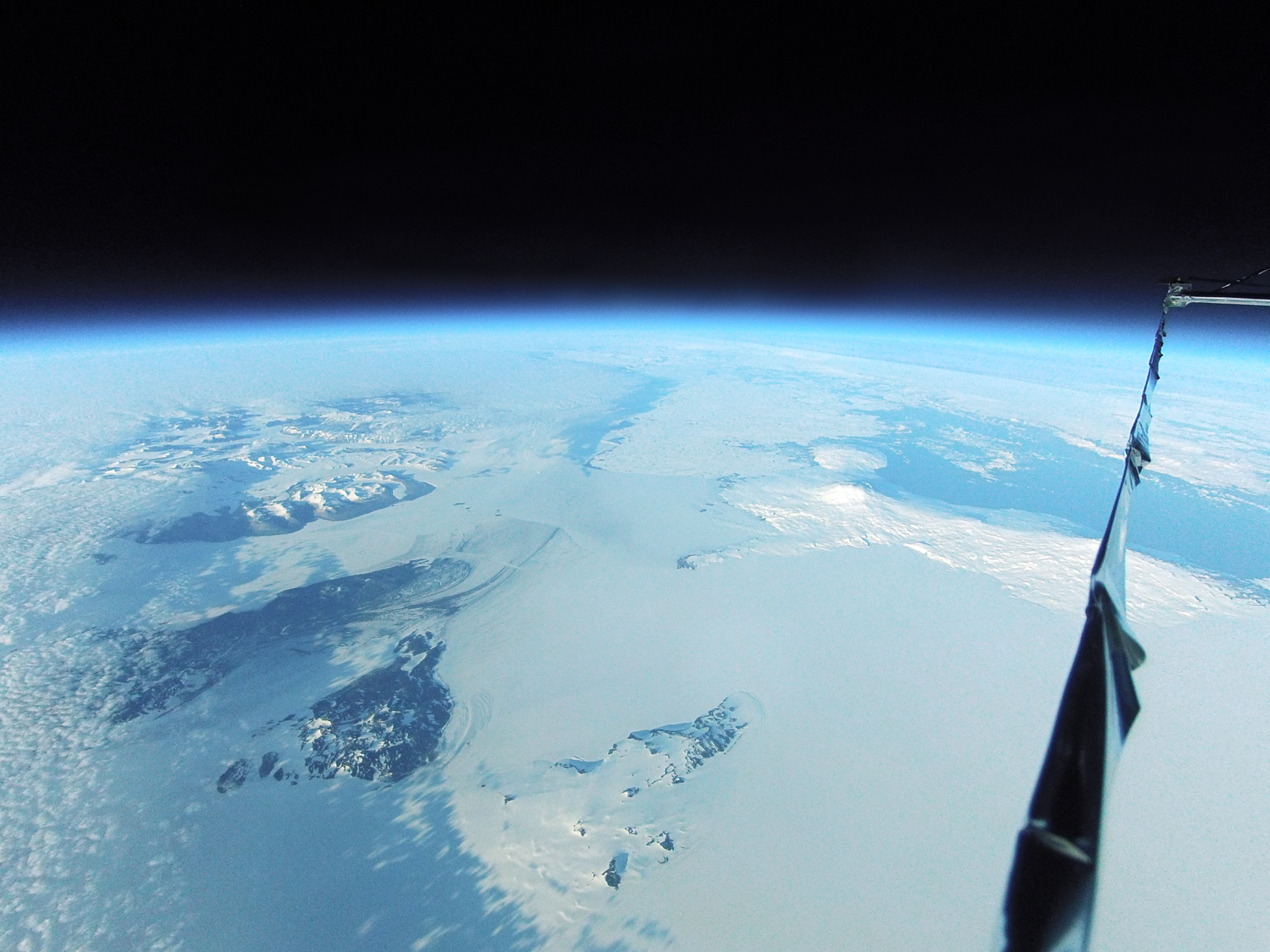
As part of an international collaboration of astrophysicists I build telescopes that operate while suspended from giant stratospheric helium balloons. These balloons can lift telescopes of > 2,500 kg up to altitudes as high as 46 km above sea level (about 4x as high as a commercial jet plane flies and above >99.5% of the Earth’s atmosphere). Balloon astronomy offers us the chance to get nearly space-quality observations, for about 1% of the cost of an equivalent satellite telescope.
For my PhD thesis project I worked on building the Balloon-borne Large Aperture Sub-mm Telescope for Polarimetry (BLASTPol). BLASTPol had a 1.8 meter aluminum primary mirror, and made maps of polarized dust emission in three different sub-mm colours (250, 350 and 500 microns). We launched BLASTPol in December 2010 and 2012, both times from Antarctica in the summer when the sun never sets and the winds in the stratosphere push the balloon telescope around the continent.
We used BLASTPol to make an incredibly detailed magnetic field map of the massive molecular cloud Vela C. By analyzing our map and comparing our observations with simulations of star formation, we have shown that in Vela C the magnetic field helps to shape the structure of the densest gas regions within the cloud, which are likely to be sites of star formation. But to understand whether magnetic fields slow down star formation in general we need observations of many more clouds.

BLAST: studying magnetic fields in star-forming regions and in nearby galaxies
I was a part of an international team of astrophysicists who developed and built the next-generation BLAST polarimeter (BLAST-TNG). BLAST-TNG mapped the sky simultaneously in three colour bands centred at 250, 350, and 500 microns using new MKID detector arrays, which result in at least a factor of 10 increase in mapping speed compared to BLASTPol.
BLAST-TNG had a short flight in January 2020, where unluckily the gondola was impacted by the falling balloon collar (which keeps the balloon from billowing out during while it is being inflated). Initially, we were able to still operate and point the telescope and verify that our new detectors were performing well. However, after 15 hours, a carbon-fibre support beam that was damaged during the launch failed and we had to end our flight after less than a day.
While the short flight was certainly disappointing our team is working to design and build an even more powerful balloon-borne observatory (The BLAST Observatory), designed to map hundreds of star-forming regions, nearby galaxies, and to study the properties of interstellar dust!
Looking at smaller scales and later stages of star and planet formation with ground-based telescopes

While telescopes on the Earth’s surface are not as sensitive as BLAST-TNG, we can build much larger telescopes on the ground. With these telescopes we can "zoom-in" to make detailed maps of magnetic fields on much smaller scales, such as dense filaments (the locations in molecular clouds where most stars form) and cores (the progenitors of individual stellar systems).
This is the goal of the Fields in Filaments survey that I am co-organizing using the TolTEC polarimeter on the 50 meter Large Millimeter Telescope in Mexico. TolTEC is a new polarimeter that is currently (as of Fall 2022) undergoing commissioning, which will have 5x better resolution than BLAST-TNG and more than 2x better resolution than any other single mm/sub-mm telescope. With the Fields in Filaments survey we will use TolTEC to map dense filaments to determine whether they are supported against gravitational collapse by magnetic fields.
I am also studying magnetic fields on even smaller scales, using the Atacama Large Millimeter Array (ALMA) a telescope in the Chilean Atacama desert that combines mm and sub-mm light from more than 40 different dishes to make maps detailed maps of individual gas cores and protoplanetary disks. By combining large scale maps from BLASTPol, detailed maps of magnetic fields in filaments with polarimeters on large ground based telescopes like TolTEC on the LMT, and maps of protostars and protoplanetary disks with ALMA, my goal is to determine how magnetic fields affect all stages of star and planet formation.
Building Balloon-borne Interferometers
Can we use balloon telescopes to make ultra-high-resolution images? The highest resolution images in astronomy to date have been made by the Event Horizon Telescope (EHT), a network of millimeter telescopes around the Earth that all simultaneously observe the same target. The EHT has managed to produce images of the shadow produced by supermassive black holes at the center of our own Milky Way galaxy and nearby M87 with an incredible resolution of 20 microarcseconds (<6 x 10-9 degrees)!
But to obtain even higher resolution maps astronomers will either have to create a network of telescopes that span distances larger than the Earth (which would require expensive space telescopes), or have to observe shorter wavelength light which is mostly absorbed by the Earth's atmosphere.
One possible solution is to use balloon telescopes as a part of this world-wide network of telescopes. Because balloon telescopes operate above most of the atmosphere they can observe at short wavelengths and they can be launched from many different locations on the Earth.
The Balloon-borne Very Large Baseline Interferometry Experiment
I am the Principle Investigator of the Balloon-borne Very Large Baseline Interferometry Experiment (BVEX) a project funded by the Canadian Space Agency to design and build a balloon-borne interferometry station.
Our goal is to demonstrate that a balloon-borne telescope can be used to generate interference fringes using a 1-meter K-band (22 GHz) balloon-borne radio telescope paired with a large ground-based radio telescope over 1000 kilometers away. We plan to launch BVEX from the Canadian Space Agency's Stratospheric Balloon Base in Timmins, Ontario in August 2025.
